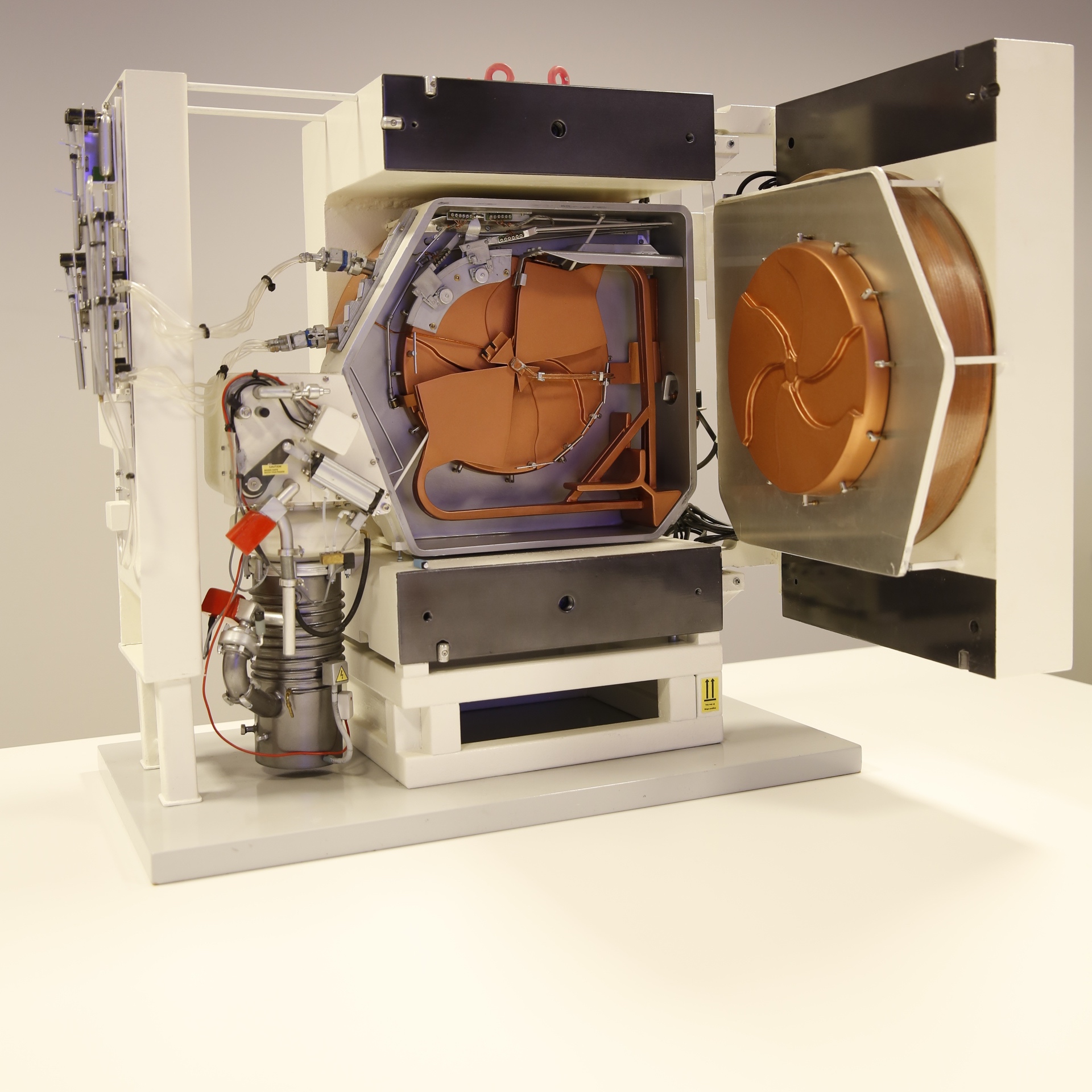
When Ernest O. Lawrence invented the cyclotron in 1932, the American physicist used his innovative particle accelerator to probe the structure of the atom. The cyclotron earned Lawrence the Nobel Prize and scientists today still use its offspring to get to the bottom of matter and even the universe itself. But the machines are also helping doctors crack the riddles of cancer and diagnose disease. “They help radiologists see the metabolic processes inside cells,” says Erik Stromqvist, general manager for cyclotrons at GE Healthcare’s plant in Uppsala, Sweden, one of the world’s largest makers of cyclotrons for the medical industry. “This is extremely important because you can tell whether the cancer is alive and whether it’s responding to treatment.”
The machines, which are as large as two telephone booths and weigh as much as 20 tons, work the same way as Lawrence’s machine. They use giant electromagnets made from tightly wound copper coils and lots of power – some 65 kilowatts – to heat up hydrogen to 5,000 degrees Celsius – almost as hot as the surface of the sun. The machines need this energy to convert the hydrogen into negatively charged hydrogen ions and accelerate them towards the speed of light.
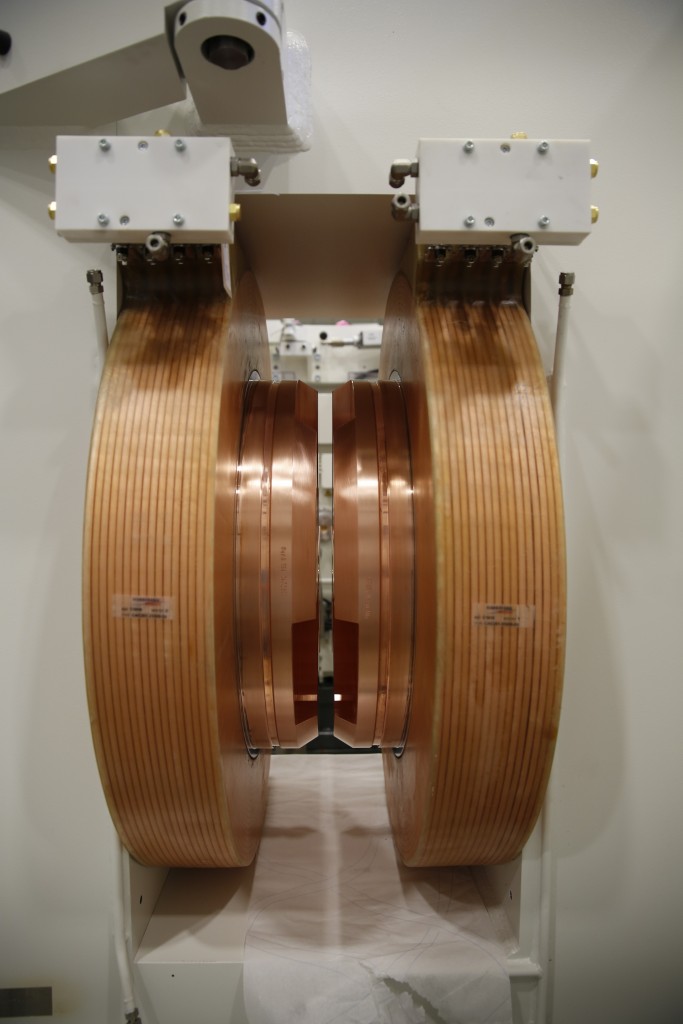
Top image: A scaled-down version of a GE cyclotron. The copper electromagnets in the center accelerate hydrogen to 20 percent of the speed of light. Above: This GE cyclotron uses cooper coils to heat up hydrogen atoms to 5,000 degrees Celsius, almost as hot as the surface of the Sun. Image credit GE Healthcare.
The ions are extracted in the center of the machine and the magnet sends them flying along a spiral trajectory. Along the way, they keep accelerating until they reach nearly 20 percent of the speed of light at the outer perimeter of the coil. There, generally speaking, they slam into oxygen atoms, knock out some of their neutrons and turn them into a radioactive isotope called fluorine-18.
Technicians then use another special GE device called FASTlab to bound the isotope, which has a half-life of less than two hours, to carrier molecules such as glucose to form fluorine-18 labelled glucose. Doctors then inject this tracer into patients and observe where it travels and whether there’s cancer inside the body.
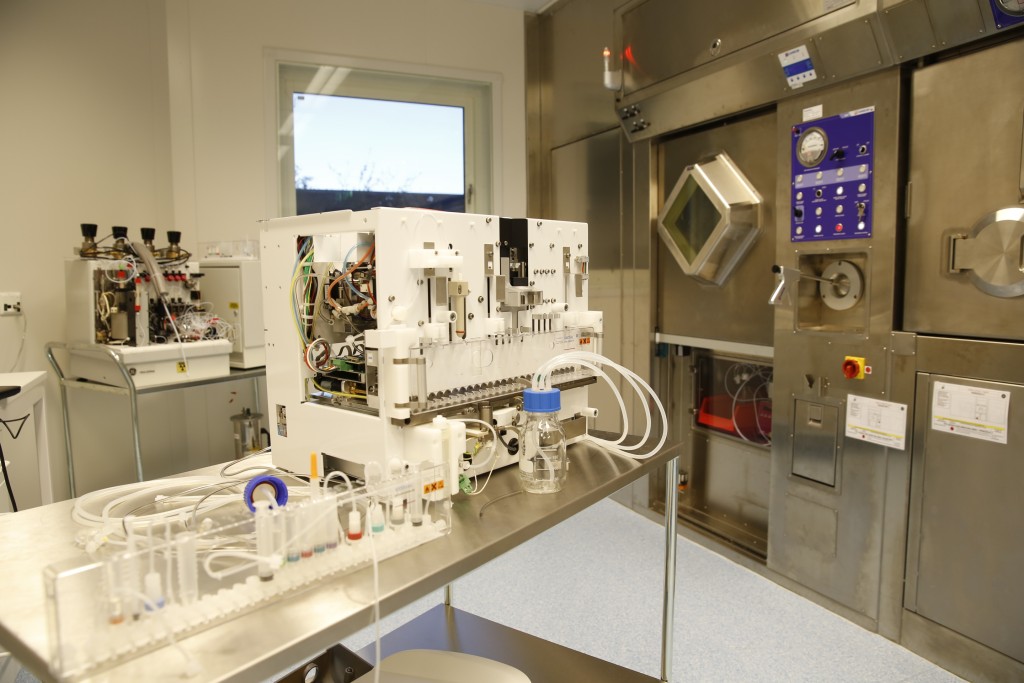
GE’s FASTlab system binds the fluorine-18 isotope, which has a half-life of less than two hours, to carrier molecules such as glucose to form fluorine-18 labelled glucose. Image credit: GE Healthcare
They can do this because active cancer needs a lot of energy to grow and consume the glucose, which is a form of sugar. “The isotope is like the world’s smallest cellphone,” Stromqvist says. “The glucose carries it to energy-hungry regions like tumors and the isotopes light them up like radio beacons.”
This type of imaging is called positron emission tomography, or PET, because the fluorine-18 isotope produces tiny particles called positrons as it decays. The positron “cell phones” generate their signal because of another bit of space science called electron-positron annihilation. The positron is the electron’s antiparticle and when it collides with an electron in the body, the pair annihilates and creates a pair of photons. Doctors use PET scanners to track these photons and see where the glucose or other carrier molecule traveled in the body.
After the procedure, all of the isotope will quickly decay and will be no longer present in the body because of its short half-life.
Unlike “anatomic imaging” using X-rays, for example, that displays the actual organs, PET helps doctors visualize what’s happening inside cells, guiding them when they are diagnosing cancer, neurological disease and other serious ailments, and even to determine whether a treatment is working. This is important for the advance of precision, or personalized medicine. “PET can help us figure out whether a cancer is treatable and whether it’s replicating,” Stromqvist says. “A tumor that’s not growing will not light up because it’s not eating as much glucose anymore.”
Why aren’t PET scanners everywhere? There are still many challenges that Stromqvist’s business is working to overcome. Since the isotopes are mildly radioactive, cyclotrons must be housed in special structures and behind 2-meter-thick walls. “Combine this with the fact that the isotope tracer has a half life of less than two hours and you have a fairly limited [geographical] area that you can serve,” Stromqvist says.
However, GE recently released a new cyclotron model the size of a large washing machine called GENtrace. This compact cyclotron – it weighs “just” 6 tons – has all the shielding built inside and allows hospitals and healthcare systems to install them pretty much anywhere. “This self-shielding cyclotron is the future of PET scanning,” Stromqvist says. “You don’t need to build a bunker around it anymore. It will simplify things.”
The GE plant in Uppsala ships between 20 and 30 cyclotrons per year. With the new machine ready, workers there are about to get busy.